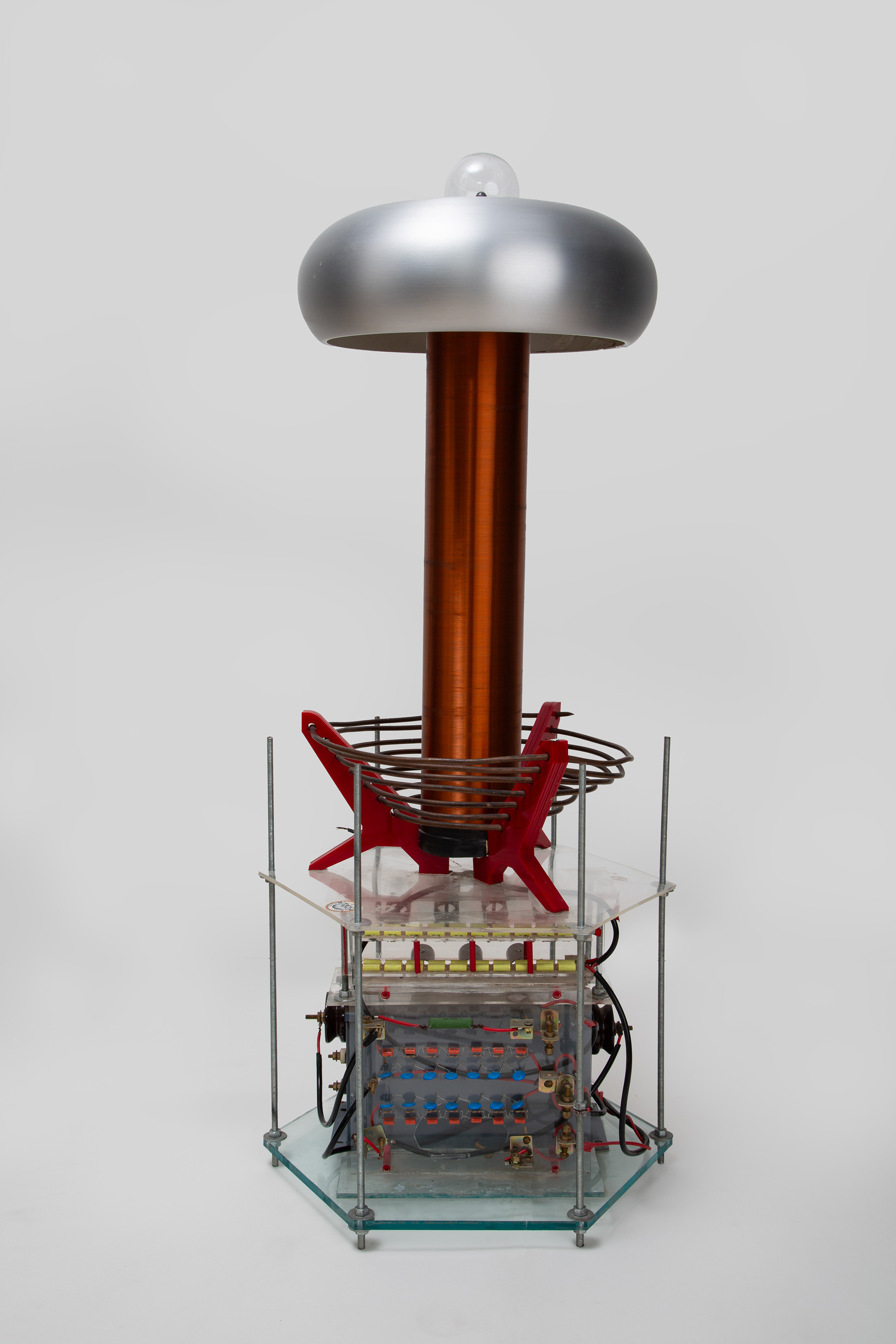
I designed and constructed a spark-gap Tesla coil, a high-voltage resonant transformer initially conceived by Nikola Tesla. The objective was to produce a device capable of generating high-frequency, high-voltage alternating currents and wireless energy transfer. Unlike modern wireless chargers that rely on close coupling and direct contact, the Tesla coil radiates energy through electromagnetic waves, enabling effects such as wireless illumination of LEDs at a distance. This approach required integrating multiple high-voltage components, careful selection of materials, and a thorough understanding of resonant frequencies, electrostatic shielding, and safety measures.
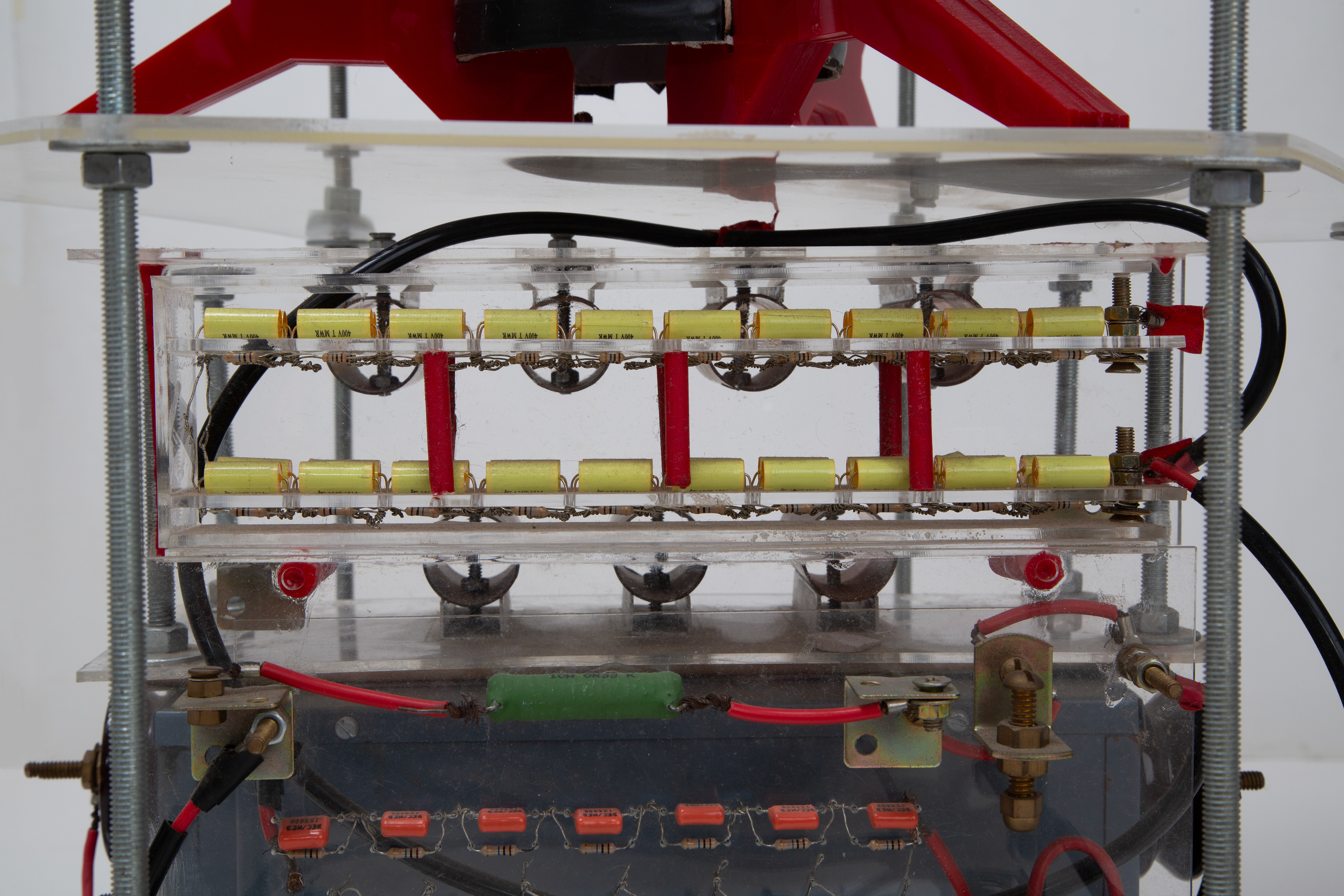
Power Supply and Primary Circuit Configuration
The primary input source was a neon sign transformer (NST) delivering approximately 15 kV at low current. This high voltage was fed through a Terry filter—comprising varistors, resistors, and capacitors—to mitigate radio frequency interference and protect the transformer from rapid transient discharges. Downstream, a static spark gap served as a voltage-controlled switch, allowing accumulated charge in a multi-module capacitor bank (MMC) to discharge into the primary coil at specific voltage thresholds. By carefully tuning this assembly, it was possible to achieve a stable and repeatable oscillatory current, with the primary coil and capacitor forming a resonant circuit at a predetermined frequency significantly higher than the transformer’s input.
Secondary Coil and Toroid Design
The secondary coil, wound from thin magnet wire on a cylindrical form, was dimensioned based on target resonant frequencies. Its length-to-diameter ratio and coil turn count were chosen to achieve resonance with the primary circuit. An aluminum toroid was a terminal electrode at the coil's top end. The toroid’s smooth, symmetrical geometry helped minimize corona formation and directed the highest field intensities outward rather than toward the coil windings or the support structure. This configuration ensured that the secondary coil achieved a high voltage step-up from the primary’s oscillations, enabling wireless energy transfer and occasional visible corona or brush discharges at the toroid’s surface.
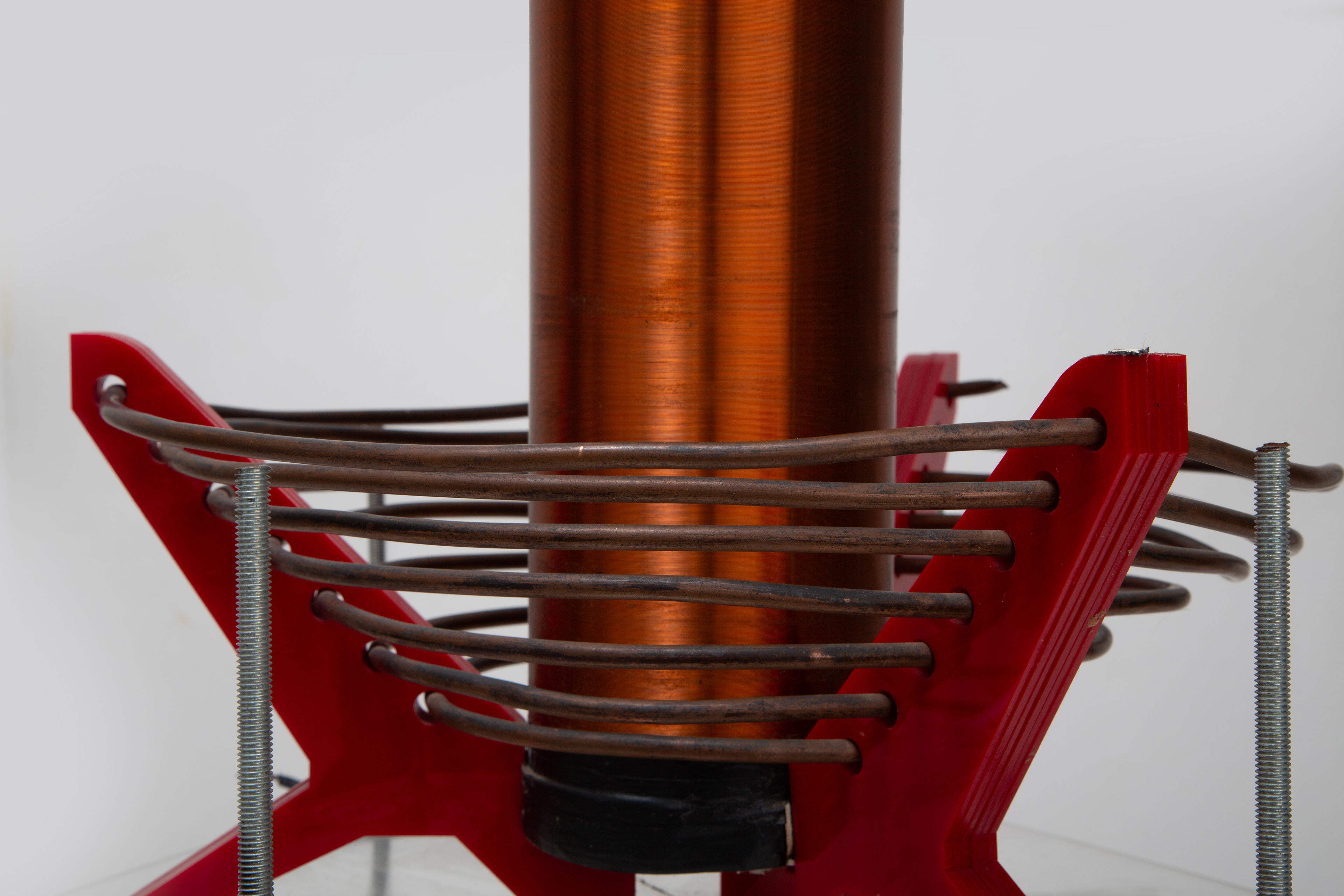
Resonance Tuning and Frequency Management
Achieving optimal performance necessitated fine-tuning the coil’s resonant frequency. Adjusting parameters involved altering the spacing of the spark gap electrodes, selecting appropriate capacitor values, and precisely positioning the primary coil tap. By monitoring the coil’s response to incremental changes—using test lamps or small antenna pickups—it was possible to converge on a configuration where the secondary coil reached maximum voltage gain. This resonant condition ensured efficient electromagnetic coupling and improved the range at which small loads (such as LED bulbs) could be powered wirelessly.
Material Selection and Construction Techniques
High-voltage operation placed strict demands on insulation quality, component spacing, and structural mechanical stability. Materials with adequate dielectric properties supported coils and capacitors, while non-conductive fixtures minimized arcing risks. Careful drilling, sanding, and deburring of metal edges reduced corona inception points, and strict wiring practices ensured minimal inductive loops and reduced radiofrequency noise. Although the coil was inherently a demonstration apparatus, considerations of mechanical robustness, ease of assembly, and component accessibility were integrated into the overall design process.
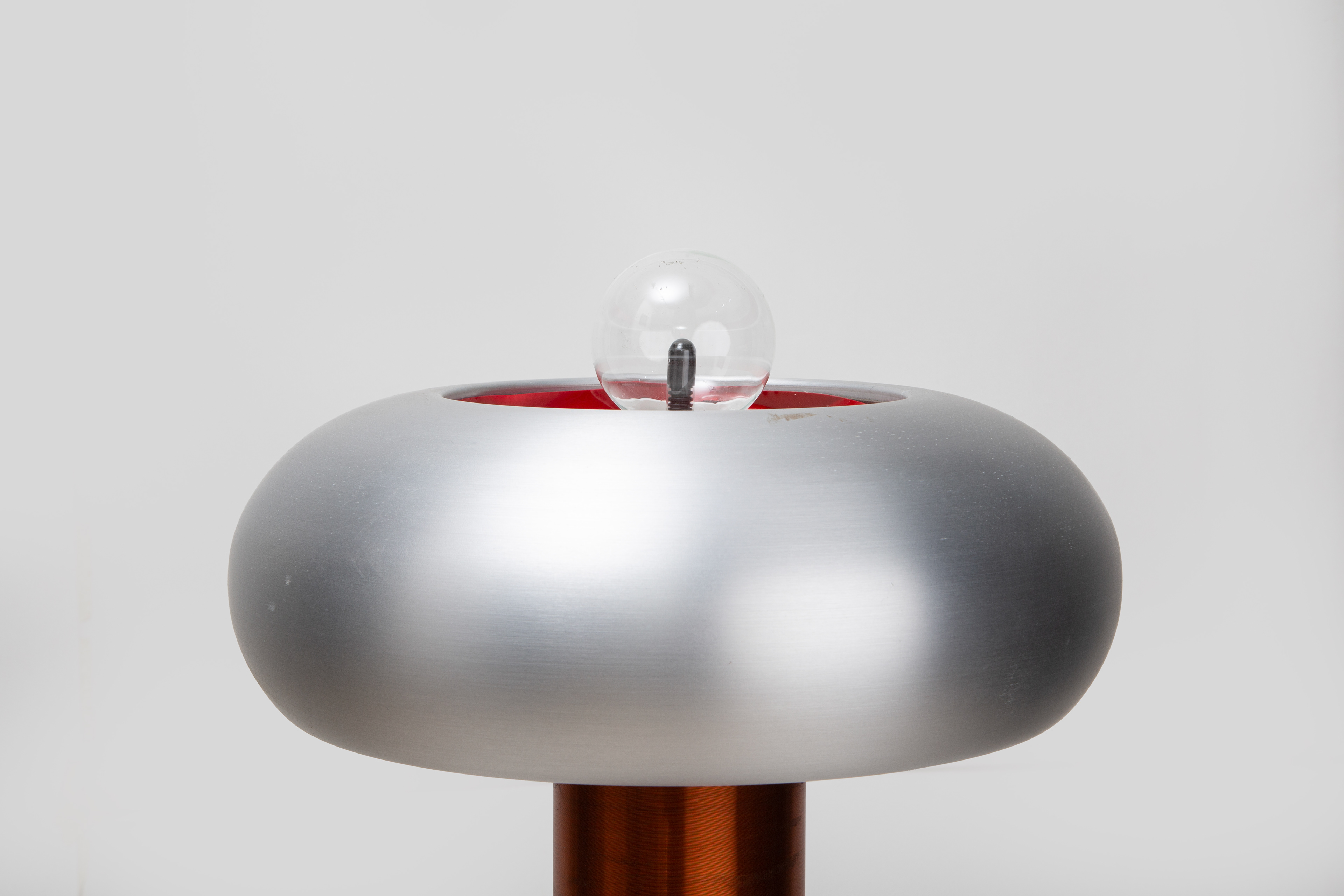
Safety Considerations and Grounding Measures
Operating at tens of kilovolts and high frequencies introduced significant safety challenges. All testing occurred with proper grounding via a dedicated RF ground connection. The Terry filter’s inclusion minimized transformer damage from backstreaming RF energy, and inter-electrode spacing in the spark gap was set to limit undesired continuous arcing. Insulation barriers and warning labels were placed to discourage direct contact with high-voltage terminals. While the Tesla coil could illuminate bulbs wirelessly, practical utility remained limited by poor coupling efficiency and the risk of uncontrolled discharges—highlighting that, despite its historical significance, the concept remains demonstrational mainly.
Validation and Performance Assessment
Controlled tests verified that the coil could generate intense oscillations and produce fields strong enough to light LEDs at distances approaching one meter.—however, fundamental physics—specifically the rapid diminishment of field strength with distance—limited efficiency. Adjusting the coil’s geometry and improving electrode smoothness reduced corona losses, but the essential inverse-square relationship and environmental factors (humidity, nearby conductive surfaces) constrained meaningful power transmission. This confirmed that, while the Tesla coil efficiently showcased high-voltage physics and electromagnetic resonance, practical wireless energy transfer at moderate ranges was not cost-effective or safe.
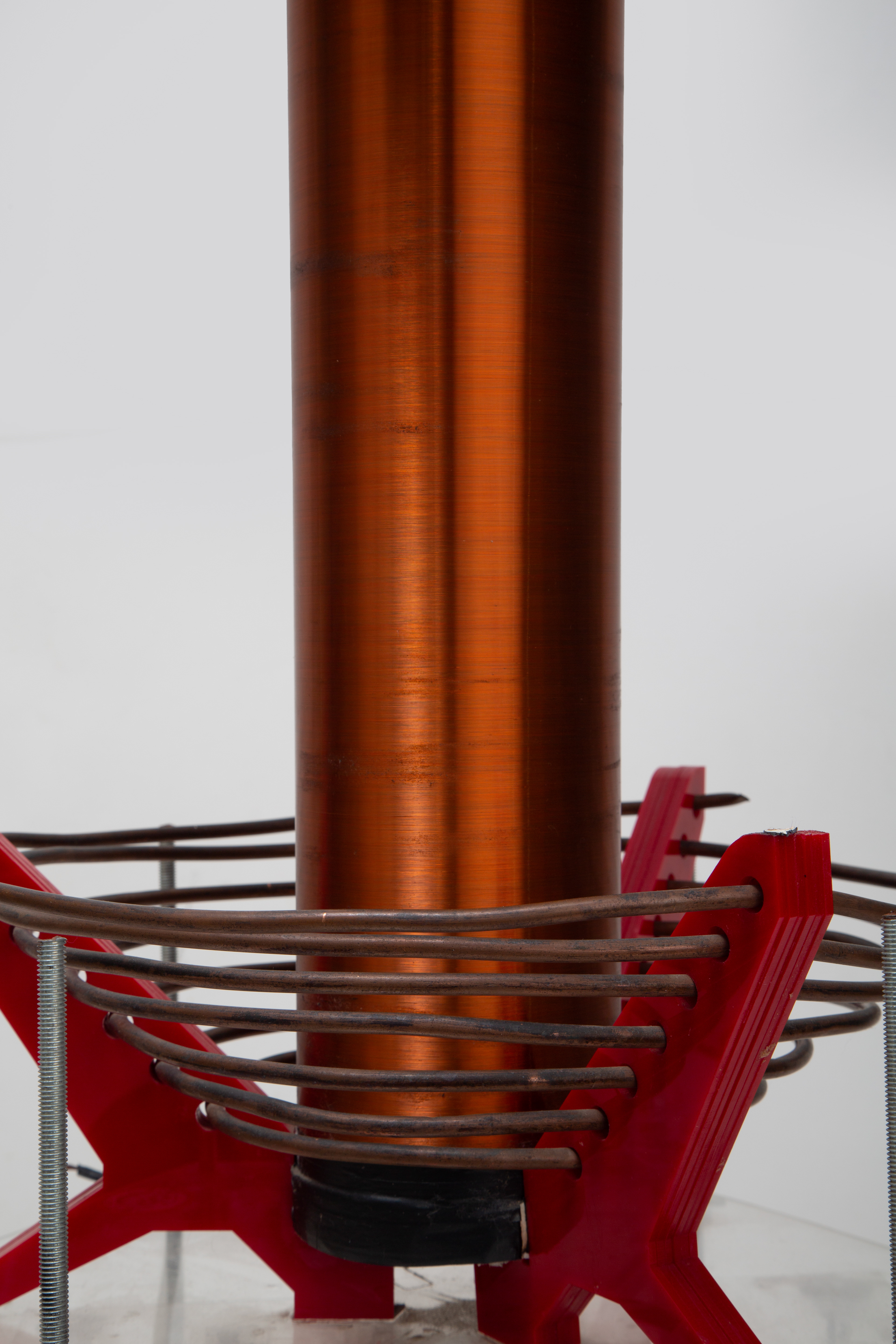
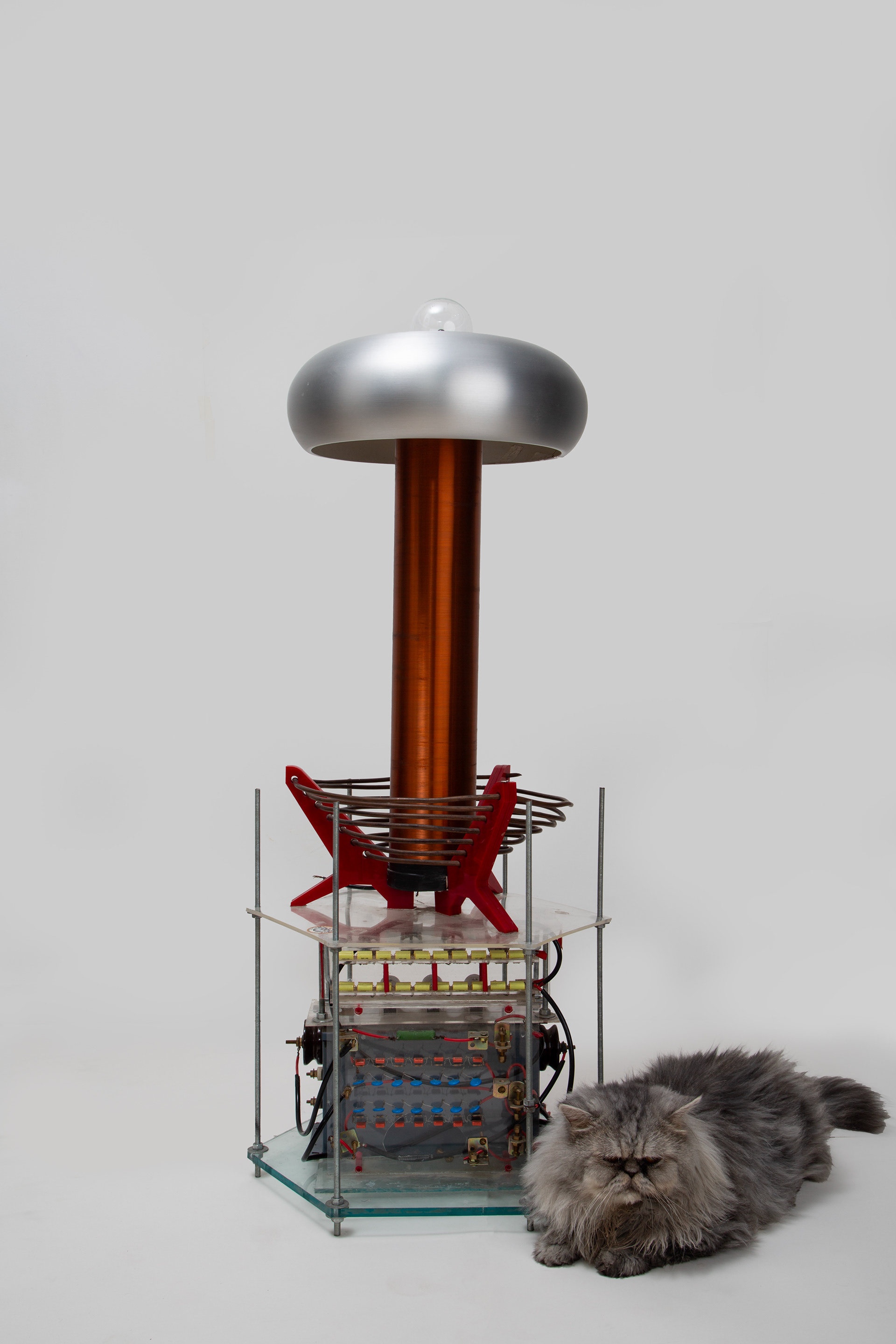
Although inherently inefficient for power distribution, the Tesla coil remains a remarkable demonstration of resonant energy transfer and early electrical engineering ingenuity. Future explorations involve solid-state drivers, improved primary-secondary coupling strategies, or more sophisticated shielding to improve safety and performance. Ultimately, this project reinforced the importance of careful design, rigorous testing, and adherence to safety protocols in high-voltage applications.
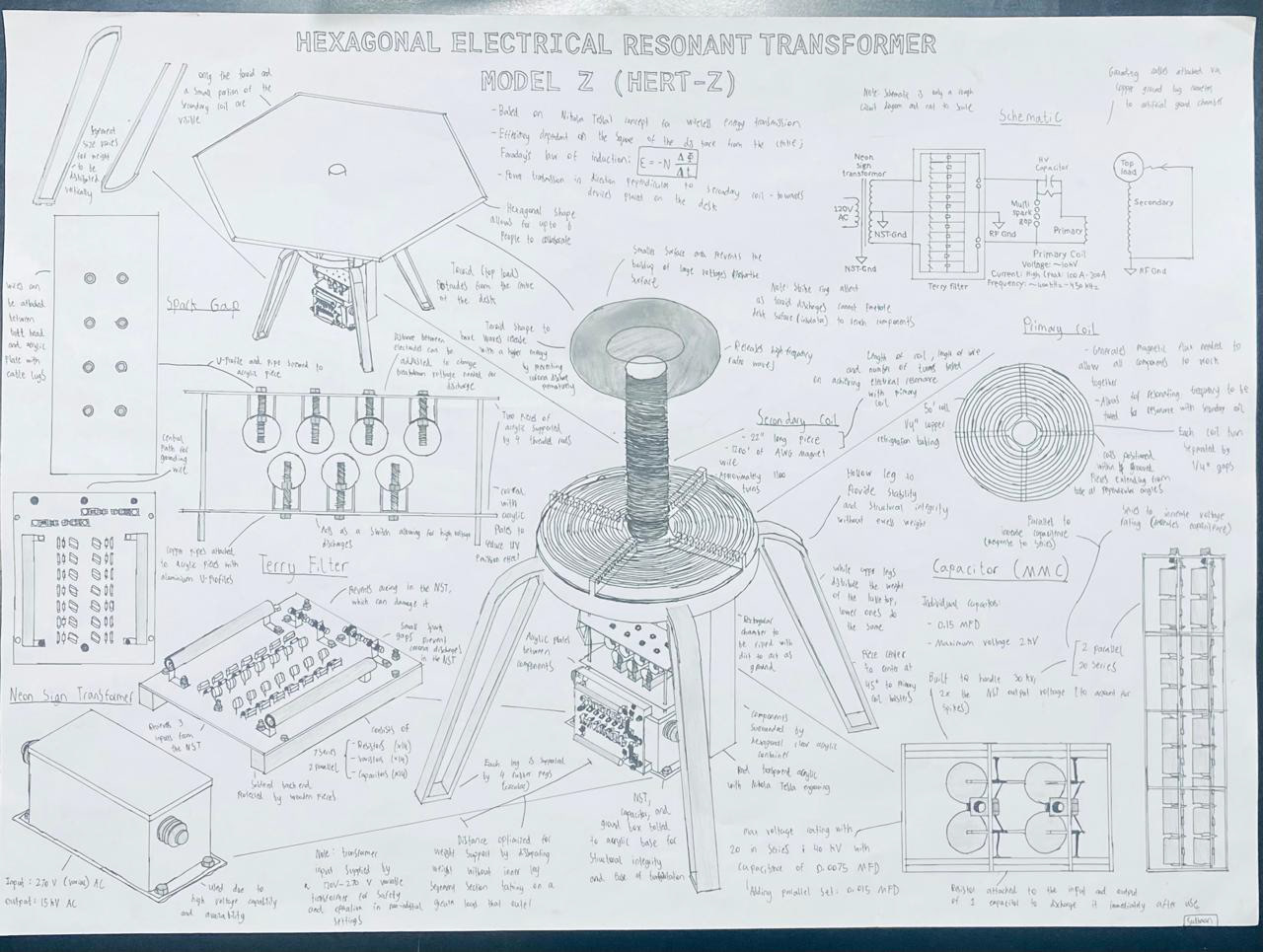
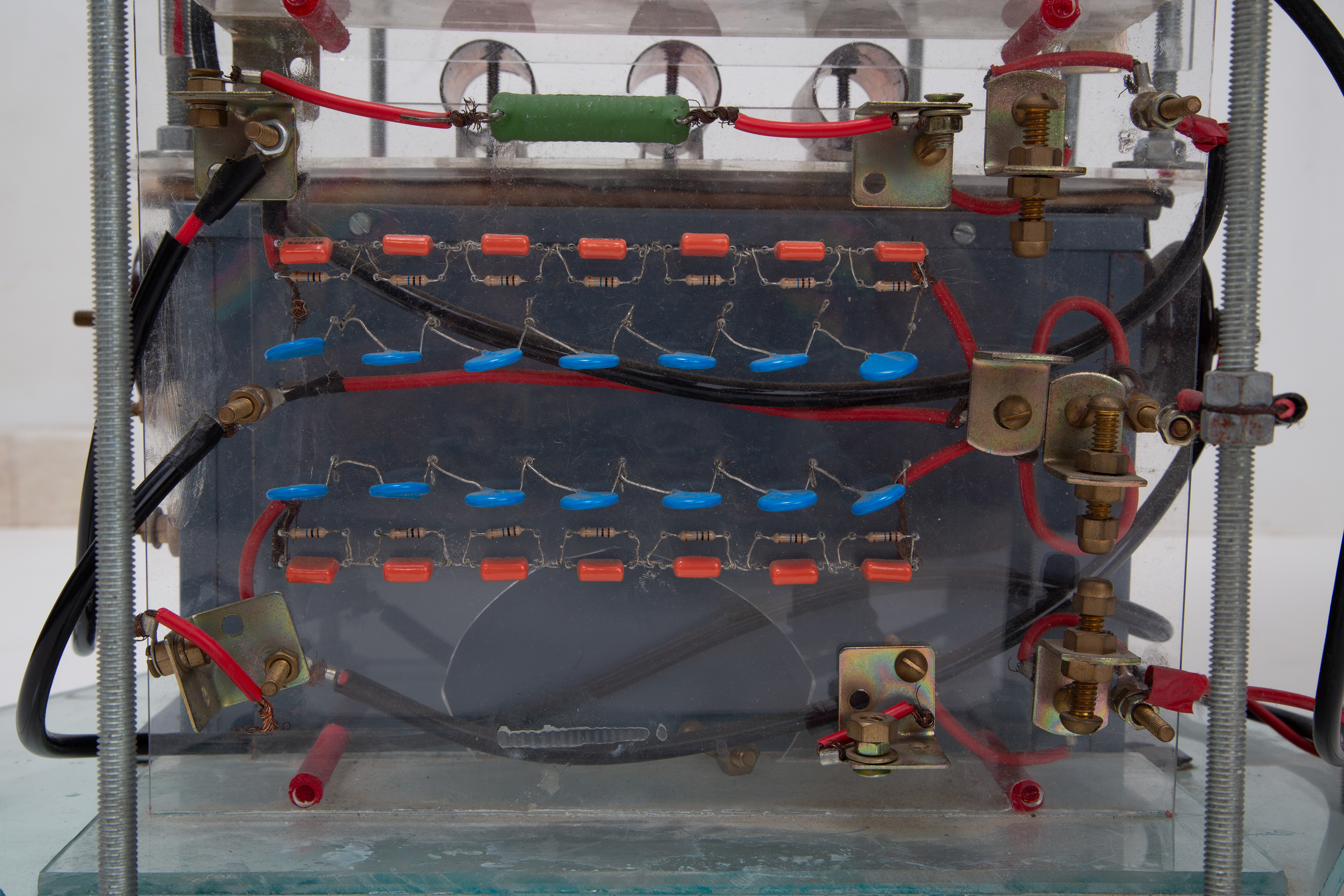
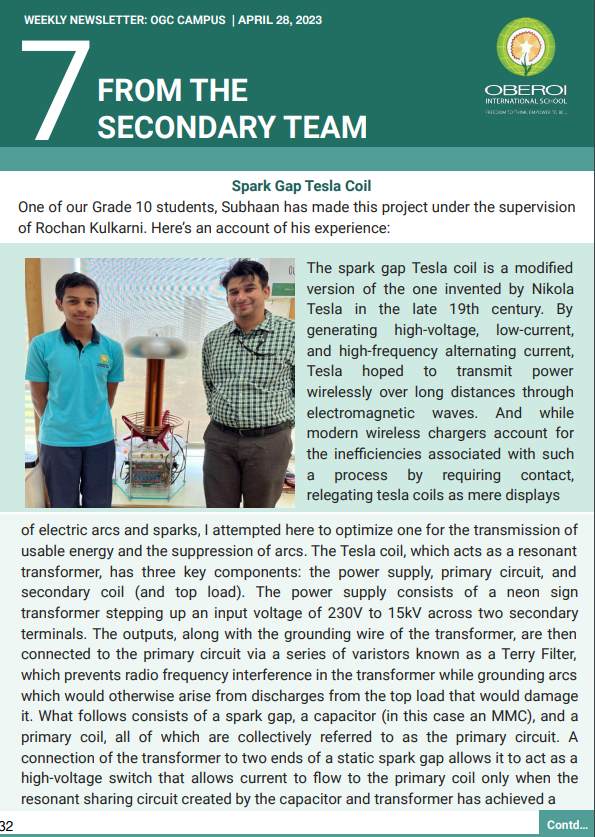